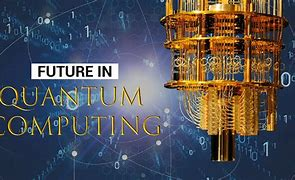
Introduction:
The future of quantum computing stands at a pivotal crossroads, poised to redefine the landscape of technology and computation. As we delve into this fascinating domain, we uncover a realm where the laws of quantum mechanics converge with computational theory, promising unprecedented advancements in speed, efficiency, and problem-solving capabilities. The journey into quantum computing is not merely about enhancing existing technologies; it is about unlocking new paradigms that challenge our fundamental understanding of computation itself.
Understanding Quantum Computing
At its core, quantum computing differs fundamentally from classical computing. Classical computers utilize bits as the smallest unit of data, which can exist in one of two states: 0 or 1. In contrast, quantum computers leverage qubits, which can exist in multiple states simultaneously due to the principle of superposition. This unique property allows quantum computers to process vast amounts of information concurrently, enabling them to tackle complex problems that are currently intractable for classical systems.
The Current State of Quantum Computing
Despite its promise, quantum computing is still in its nascent stages. Recent advancements have led to the development of functional quantum processors capable of executing specific tasks, such as optimization problems and quantum simulations. However, significant challenges remain, particularly concerning the stability and scalability of quantum hardware, the development of robust quantum algorithms, and effective error correction techniques. These hurdles must be addressed to transition quantum computing from experimental setups to practical, widespread applications.
Future Directions in Quantum Computing
The trajectory of quantum computing is marked by several promising avenues of exploration:
1. Enhanced Quantum Hardware
Improving the reliability and performance of quantum hardware is critical. Researchers are focused on developing more stable quantum processors and advanced error correction methods to mitigate the effects of noise and decoherence, which can disrupt quantum computations. Achieving a sufficient number of high-quality qubits is essential for practical quantum advantage, often referred to as quantum supremacy.
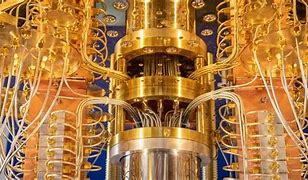
2. Breakthroughs in Chemistry and Materials Science
Quantum computing holds the potential to revolutionize fields such as chemistry and materials science by simulating complex molecular interactions that are impossible to model with classical computers. This capability could accelerate the discovery of new materials and drugs, significantly impacting industries ranging from pharmaceuticals to energy.
3. Advancements in Cryptography
The rise of quantum computing poses both a threat and an opportunity for cryptography. While quantum computers could potentially break widely used encryption methods, they also pave the way for the development of quantum-safe encryption techniques that are resistant to quantum attacks. This duality highlights the need for a proactive approach to cybersecurity in the quantum era.
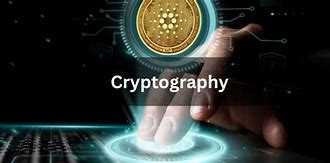
4. Optimization and Machine Learning
Quantum computing is particularly well-suited for solving optimization problems, which are prevalent in logistics, finance, and supply chain management. Additionally, the integration of quantum computing with machine learning could lead to significant advancements in data analysis and pattern recognition, enhancing the capabilities of artificial intelligence systems.
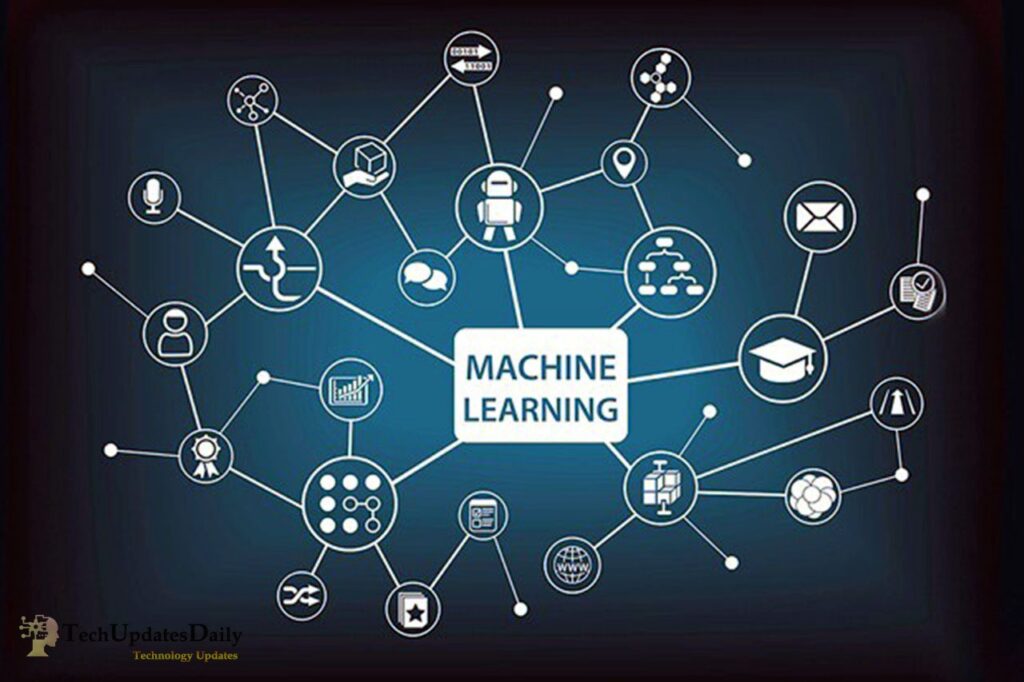
5. Hybrid Classical-Quantum Systems
Many future applications may require a hybrid approach that combines classical and quantum computing. Researchers are exploring methods to integrate classical algorithms with quantum processes, leveraging the strengths of both paradigms to achieve optimal results in various applications.
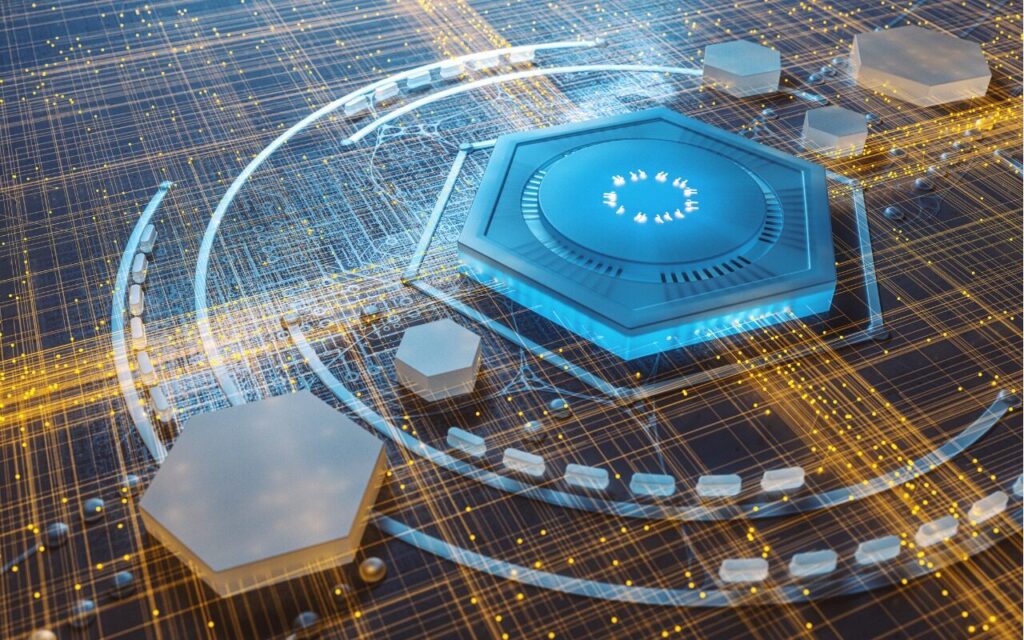
The Quantum Internet and Networking
As quantum computing evolves, the concept of a quantum internet emerges, enabling the transmission of quantum information across networks. This quantum network would facilitate parallel computations by connecting multiple quantum computers, enhancing computational speeds and capabilities. However, the implementation of such networks is still in its infancy, with significant technical challenges to overcome, particularly concerning noise and the preservation of quantum states during transmission.
What are the biggest challenges facing the development of quantum computing?
The development of quantum computing is fraught with significant challenges that hinder its progression from theoretical concepts to practical applications. As researchers and engineers strive to unlock the full potential of this revolutionary technology, they face a multitude of obstacles that must be addressed. Here are the most pressing challenges:
1. Quantum Decoherence
Quantum decoherence is a fundamental issue that arises from the fragile nature of qubits, the building blocks of quantum computers. Qubits can lose their quantum properties due to interactions with their environment, leading to errors in calculations. This loss of coherence limits the time during which quantum information can be processed, making it crucial to develop techniques that enhance the stability and longevity of qubits.
2. Error Correction
Error correction is often cited as the most critical challenge in quantum computing. Due to the sensitivity of qubits to noise and environmental disturbances, errors can accumulate rapidly during computations. Developing robust quantum error correction methods is essential to ensure reliable and accurate quantum computations. However, these techniques require additional qubits and complex encoding schemes, complicating the overall architecture of quantum systems.
3. Scalability
Scaling quantum computers to accommodate a larger number of qubits while maintaining coherence and low error rates is a significant hurdle. Current quantum systems are relatively small compared to classical computers, and increasing the number of qubits introduces additional challenges related to error rates and control mechanisms. Researchers are exploring various qubit technologies, such as superconducting qubits and trapped ions, to achieve the necessary scalability for practical applications.
4. Hardware Development
The development of high-quality quantum hardware is crucial for the advancement of quantum computing. This includes not only the qubits themselves but also the control electronics and systems that support them. Each qubit technology has its own strengths and weaknesses, and creating a scalable, fault-tolerant qubit technology remains a primary focus of research.
5. Software Development
Quantum algorithms and programming languages are still in their early stages of development. Unlike classical algorithms, quantum algorithms require a different approach to problem-solving, which presents a challenge for software developers. There is a pressing need for new programming languages, compilers, and optimization tools that can effectively harness the capabilities of quantum computers.
6. Classical Computer Interfaces
Quantum computers are not intended to replace classical computers but to complement them. Therefore, developing efficient interfaces for data transfer between classical and quantum systems is essential for practical applications. This integration is crucial for leveraging the strengths of both computing paradigms.
7. Standards and Protocols
As quantum computing matures, the establishment of standards and protocols for hardware, software, and communication interfaces becomes vital. These standards will ensure compatibility and interoperability between different quantum computing platforms, facilitating broader adoption and collaboration within the field.
8. Trained Talent
The quantum computing field faces a shortage of skilled professionals. The number of individuals trained in quantum technologies is limited, creating a bottleneck in the workforce necessary to advance research and development. This challenge is compounded by the need for practical quantum computers to attract more talent into the field.
9. Overall Expense
The cost associated with quantum computing remains a significant barrier. The development of quantum hardware, the recruitment of specialized talent, and the establishment of complex supply chains all contribute to high expenses. Finding sustainable investments and managing these costs will be essential for the continued growth of quantum computing.
What are the key differences between superconducting qubits and trapped ion qubits?
The key differences between superconducting qubits and trapped ion qubits are:
Qubit Technology
- Superconducting qubits use phenomena found in electric superconducting circuits to create a quantum two-state system.
- Trapped ion qubits literally trap ions (charged atoms) using magnetic fields and hold them in place, with the outermost electron orbiting the nucleus used as the qubit.
Gate Speed
- Superconducting qubits have faster gate times (faster operation times) compared to trapped ion qubits.
- Trapped ion qubits are generally significantly slower than their superconducting counterparts.
Decoherence Time
- Trapped ion qubits have much longer decoherence times (qubit memory) than superconducting qubits.
- Superconducting qubits have fast decoherence times, meaning their ‘memory’ is very short-lived.
Connectivity
- Trapped ion qubits can be reconfigured, allowing each qubit to interact with every other qubit in the computer.
- Superconducting qubits can normally only interact with a handful of neighboring qubits on the device.
Operating Temperature
- Trapped ion qubits can operate at around 4K (four degrees above absolute zero), which is much cheaper and easier than the 0.1K needed by superconducting qubits.
- Superconducting qubits must be kept very cold, below 100mK (0.1 degrees above absolute zero), which can be expensive and inconvenient.
Scalability
- Superconducting qubits can take advantage of existing methods and processes, making it easier to envision a scalable quantum computer.
- Trapped ion qubits require more technological improvements before a scalable system can be imagined.
FAQs
Here are some commonly asked questions about quantum computing and their answers:
What is a quantum computer?
A quantum computer is a device that performs computations by manipulating quantum mechanical phenomena, such as superposition and entanglement. Unlike classical computers that use bits representing 0 or 1, quantum computers use quantum bits or qubits that can be in a superposition of both 0 and 1 states simultaneously.
Why all the effort to build quantum computers?
Quantum computers have the potential to solve certain problems much faster than classical computers. These include problems in cryptography, optimization, quantum chemistry simulations, and machine learning. Quantum computers could break many of the encryption schemes used today and enable new discoveries in materials science and drug development.
What are the main challenges in building quantum computers?
The main challenges include:
- Quantum decoherence: Qubits are very fragile and can lose their quantum properties due to interactions with the environment, leading to errors. Maintaining qubits in a coherent state is difficult.
- Scalability: Building quantum computers with a large number of reliable qubits is challenging. As the number of qubits increases, so do the chances of errors.
- Error correction: Quantum errors are different from classical errors and require new error correction techniques. Implementing efficient quantum error correction is crucial for reliable computations.
- Software development: Quantum algorithms and programming languages are still in early stages. Developing efficient quantum software is a significant challenge.
When will quantum computers be useful?
Quantum computers are still in the research and development phase. While some quantum computers can already outperform classical computers on specific tasks, it will likely take several more years before quantum computers can provide a practical advantage over classical computers for real-world applications. Estimates suggest that quantum advantage for practical applications may be achieved by 2030-2040.
Will quantum computers replace classical computers?
No, quantum computers are not expected to replace classical computers. Instead, they will complement classical computers by solving certain problems much faster. Classical computers will still be needed for many tasks, and the two paradigms will likely work together in hybrid systems.
What are some potential applications of quantum computing?
Potential applications include:
- Cryptography: Breaking many of the encryption schemes used today
- Optimization: Solving complex optimization problems faster
- Quantum chemistry simulations: Simulating chemical reactions and materials
- Machine learning: Speeding up certain machine learning algorithms
- Financial modeling: Pricing financial instruments and managing risk
- Drug discovery: Accelerating the discovery of new drugs
What are some of the companies working on quantum computing?
Many major tech companies are investing in quantum computing research and development, including IBM, Google, Microsoft, Amazon, Intel, Alibaba, and Baidu. There are also several startups focused on quantum computing, such as IonQ, Rigetti Computing, and PsiQuantum.
How can I learn more about quantum computing?
There are many online resources available to learn about quantum computing, including:
- Quantum Computing Report: https://quantumcomputingreport.com/
- Quantum Computing Stack Exchange: https://quantumcomputing.stackexchange.com/
- Quantum Computing Courses on Coursera and edX
- Books like “Quantum Computing: A Gentle Introduction” by Michael A. Nielsen and Isaac L. Chuang.
Read Related Post
Understanding the Concept of Cybersecurity Enhancement